Radio astronomy
Radio astronomy is a subfield of
Radio astronomy is conducted using large radio antennas referred to as radio telescopes, that are either used singularly, or with multiple linked telescopes utilizing the techniques of radio interferometry and aperture synthesis. The use of interferometry allows radio astronomy to achieve high angular resolution, as the resolving power of an interferometer is set by the distance between its components, rather than the size of its components.
Radio astronomy differs from radar astronomy in that the former is a passive observation (i.e., receiving only) and the latter an active one (transmitting and receiving).
History
Before Jansky observed the Milky Way in the 1930s, physicists speculated that radio waves could be observed from astronomical sources. In the 1860s, James Clerk Maxwell's equations had shown that electromagnetic radiation is associated with electricity and magnetism, and could exist at any wavelength. Several attempts were made to detect radio emission from the Sun including an experiment by German astrophysicists Johannes Wilsing and Julius Scheiner in 1896 and a centimeter wave radiation apparatus set up by Oliver Lodge between 1897 and 1900. These attempts were unable to detect any emission due to technical limitations of the instruments. The discovery of the radio reflecting ionosphere in 1902, led physicists to conclude that the layer would bounce any astronomical radio transmission back into space, making them undetectable.[1]
Jansky announced his discovery at a meeting in Washington, D.C., in April 1933 and the field of radio astronomy was born.
After 1935, Jansky wanted to investigate the radio waves from the Milky Way in further detail, but Bell Labs reassigned him to another project, so he did no further work in the field of astronomy. His pioneering efforts in the field of radio astronomy have been recognized by the naming of the fundamental unit of
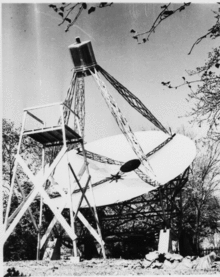
Grote Reber was inspired by Jansky's work, and built a parabolic radio telescope 9m in diameter in his backyard in 1937. He began by repeating Jansky's observations, and then conducted the first sky survey in the radio frequencies.[12] On February 27, 1942, James Stanley Hey, a British Army research officer, made the first detection of radio waves emitted by the Sun.[13] Later that year George Clark Southworth,[14] at Bell Labs like Jansky, also detected radiowaves from the Sun. Both researchers were bound by wartime security surrounding radar, so Reber, who was not, published his 1944 findings first.[15] Several other people independently discovered solar radio waves, including E. Schott in Denmark[16] and Elizabeth Alexander working on Norfolk Island.[17][18][19][20]

At
Techniques
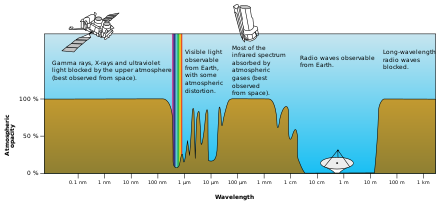
Radio astronomers use different techniques to observe objects in the radio spectrum. Instruments may simply be pointed at an energetic radio source to analyze its emission. To "image" a region of the sky in more detail, multiple overlapping scans can be recorded and pieced together in a mosaic image. The type of instrument used depends on the strength of the signal and the amount of detail needed.
Observations from the
Radio telescopes
Radio telescopes may need to be extremely large in order to receive signals with low
Radio interferometry



The difficulty in achieving high resolutions with single radio telescopes led to radio
The Cambridge group of Ryle and Vonberg observed the Sun at 175 MHz for the first time in mid July 1946 with a Michelson interferometer consisting of two radio antennas with spacings of some tens of meters up to 240 meters. They showed that the radio radiation was smaller than 10
Modern
Very-long-baseline interferometry
Beginning in the 1970s, improvements in the stability of radio telescope receivers permitted telescopes from all over the world (and even in Earth orbit) to be combined to perform
The pre-eminent VLBI arrays operating today are the Very Long Baseline Array (with telescopes located across North America) and the European VLBI Network (telescopes in Europe, China, South Africa and Puerto Rico). Each array usually operates separately, but occasional projects are observed together producing increased sensitivity. This is referred to as Global VLBI. There are also a VLBI networks, operating in Australia and New Zealand called the LBA (Long Baseline Array),[22] and arrays in Japan, China and South Korea which observe together to form the East-Asian VLBI Network (EAVN).[23]
Since its inception, recording data onto hard media was the only way to bring the data recorded at each telescope together for later correlation. However, the availability today of worldwide, high-bandwidth networks makes it possible to do VLBI in real time. This technique (referred to as e-VLBI) was originally pioneered in Japan, and more recently adopted in Australia and in Europe by the EVN (European VLBI Network) who perform an increasing number of scientific e-VLBI projects per year.[24]
Astronomical sources
Radio astronomy has led to substantial increases in astronomical knowledge, particularly with the discovery of several classes of new objects, including pulsars, quasars[25] and radio galaxies. This is because radio astronomy allows us to see things that are not detectable in optical astronomy. Such objects represent some of the most extreme and energetic physical processes in the universe.
The
Other sources include:
- Sun
- Jupiter
- Sagittarius A, the Galactic Center of the Milky Way, with one portion Sagittarius A* thought to be a radio wave emitting supermassive black hole
- Active galactic nuclei and pulsars have jets of charged particles which emit synchrotron radiation
- Merging galaxy clusters often show diffuse radio emission[26]
- Supernova remnants can also show diffuse radio emission; pulsars are a type of supernova remnant that shows highly synchronous emission.
- The blackbodyradio/microwave emission
International regulation
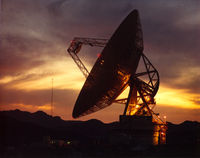

Radio astronomy service (also: radio astronomy radiocommunication service) is, according to Article 1.58 of the
Frequency allocation
The allocation of radio frequencies is provided according to Article 5 of the ITU Radio Regulations (edition 2012).[28]
In order to improve harmonisation in spectrum utilisation, the majority of service-allocations stipulated in this document were incorporated in national Tables of Frequency Allocations and Utilisations which is with-in the responsibility of the appropriate national administration. The allocation might be primary, secondary, exclusive, and shared.
- primary allocation: is indicated by writing in capital letters (see example below)
- secondary allocation: is indicated by small letters
- exclusive or shared utilization: is within the responsibility of administrations
In line to the appropriate
Allocation to services | ||
Region 1 | Region 2 | Region 3 |
13 360–13 410 kHz FIXED
| ||
25 550–25 650 RADIO ASTRONOMY | ||
37.5–38.25 MHz FIXED
| ||
322–328.6 FIXED
| ||
406.1–410 FIXED
| ||
1 400–1 427 EARTH EXPLORATION-SATELLITE (passive)
| ||
1 610.6–1 613.8 MOBILE-SATELLITE
RADIO ASTRONOMY
|
1 610.6–1 613.8 MOBILE-SATELLITE
RADIO ASTRONOMY
RADIODETERMINATION-
|
1 610.6–1 613.8 MOBILE-SATELLITE
RADIO ASTRONOMY
Radiodetermination-
|
10.6–10.68 GHz RADIO ASTRONOMY and other services | ||
10.68–10.7 RADIO ASTRONOMY and other services | ||
14.47–14.5 RADIO ASTRONOMY and other services | ||
15.35–15.4 RADIO ASTRONOMY and other services | ||
22.21–22.5 RADIO ASTRONOMY and other services | ||
23.6–24 RADIO ASTRONOMY and other services | ||
31.3–31.5 RADIO ASTRONOMY and other services |
See also
- Atacama Large Millimeter Array
- Channel 37
- Gamma-ray astronomy
- Infrared astronomy
- Radar astronomy
- Time smearing
- X-ray astronomy
- Waves (Juno) (radio instrument on the Juno Jupiter orbiter)
- Radio Galaxy Zoo
- Würzburg radar#Post-war use in astronomy
References
- ^ F. Ghigo. "Pre-History of Radio Astronomy". National Radio Astronomy Observatory. Archived from the original on 2020-06-15. Retrieved 2010-04-09.
- ^ a b World of Scientific Discovery on Karl Jansky. Archived from the original on 2012-01-21. Retrieved 2010-04-09.
- S2CID 4063838.
- ^ Hirshfeld, Alan (2018). "Karl Jansky and the Discovery of Cosmic Radio Waves". aas.org. American Astronomical Society. Archived from the original on 29 September 2021. Retrieved 21 September 2021.
In April 1933, closing in on nearly two years of study, Jansky read his breakthrough paper, "Electrical Disturbances Apparently of Extraterrestrial Origin," before a meeting of the International Scientific Radio Union in Washington, DC. The strongest of the extraterrestrial waves, he found, emanate from a region in Sagittarius centered around right ascension 18 hours and declination — 20 degrees — in other words, from the direction of the galactic center. Jansky's discovery made the front page of the New York Times on 5 May 1933, and the field of radio astronomy was born.
- S2CID 47549559. along with an explanatory preface by W.A. Imbriale, Introduction To "Electrical Disturbances Apparently Of Extraterrestrial Origin".
- S2CID 51632813.
- ISBN 978-3-527-40764-4.
- ISBN 978-0-387-85355-0.
- S2CID 1431308.
- doi:10.1086/160401.
- ^ "This Month in Physics History May 5, 1933: The New York Times Covers Discovery of Cosmic Radio Waves". aps.org. American Physical Society (May 2015) Volume 24, Number 5. Archived from the original on 14 September 2021. Retrieved 21 September 2021.
Jansky died in 1950 at the age of 44, the result of a massive stroke stemming from his kidney disease. When that first 1933 paper was reprinted in Proceedings of the IEEE in 1984, the editors noted that Jansky's work would mostly likely have won a Nobel prize, had the scientist not died so young. Today the "jansky" is the unit of measurement for radio wave intensity (flux density).
- ^ "Grote Reber". Archived from the original on 2020-08-07. Retrieved 2010-04-09.
- ISBN 978-0080187617.
- .
- Bibcode:1999ApJ...525C.371K.
- .
- ^ Alexander, F.E.S. (1945). Long Wave Solar Radiation. Department of Scientific and Industrial Research, Radio Development Laboratory.
- Bibcode:1945rdlr.book.....A.
- ^ Alexander, F.E.S. (1946). "The Sun's radio energy". Radio & Electronics. 1 (1): 16–17. (see R&E holdings at NLNZ Archived 2016-07-23 at archive.today.)
- ISBN 978-1-4020-3723-8.
- ^ "Radio Astronomy". Cambridge University: Department of Physics. Archived from the original on 2013-11-10.
- ^ "VLBI at the ATNF". 7 December 2016. Archived from the original on 1 May 2021. Retrieved 16 June 2015.
- ^ "East Asia VLBI Network and Asia Pacific Telescope". Archived from the original on 2021-04-28. Retrieved 2015-06-16.
- ^ "A technological breakthrough for radio astronomy – Astronomical observations via high-speed data link". 26 January 2004. Archived from the original on 2008-12-03. Retrieved 2008-07-22.
- from the original on 12 September 2009. Retrieved 3 October 2014.
- ^ "Conclusion". Archived from the original on 2006-01-28. Retrieved 2006-03-29.
- ^ ITU Radio Regulations, Section IV. Radio Stations and Systems – Article 1.58, definition: radio astronomy service / radio astronomy radiocommunication service
- ^ ITU Radio Regulations, CHAPTER II – Frequencies, ARTICLE 5 Frequency allocations, Section IV – Table of Frequency Allocations
Further reading
- Journals
- Gart Westerhout (1972). "The early history of radio astronomy". S2CID 56034495.
- Hendrik Christoffel van de Hulst (1945). "Radiostraling uit het wereldruim. II. Herkomst der radiogolven". Nederlands Tijdschrift voor Natuurkunde (in Dutch). 11: 210–221.
- Books
- Gerrit Verschuur The Invisible Universe: The Story of Radio Astronomy Springer 2015
- Bruno Bertotti (ed.), Modern Cosmology in Retrospect. Cambridge University Press 1990.
- James J. Condon, et al.: Essential Radio Astronomy. Princeton University Press, Princeton 2016, ISBN 9780691137797.
- Robin Michael Green, Spherical Astronomy. Cambridge University Press, 1985.
- Raymond Haynes, Roslynn Haynes, and Richard McGee, Explorers of the Southern Sky: A History of Australian Astronomy. Cambridge University Press 1996.
- J.S. Hey, The Evolution of Radio Astronomy. Neale Watson Academic, 1973.
- David L. Jauncey, Radio Astronomy and Cosmology. Springer 1977.
- Roger Clifton Jennison, Introduction to Radio Astronomy. 1967.
- Jobn D. Kraus, Martt; E. Tiuri, and Antti V. Räisänen, Radio Astronomy, 2nd ed, Cygnus-Quasar Books, 1986.
- Albrecht Krüger, Introduction to Solar Radio Astronomy and Radio Physics. Springer 1979.
- David P.D. Munns, A Single Sky: How an International Community Forged the Science of Radio Astronomy. Cambridge, MA: MIT Press, 2013.
- Allan A. Needell, Science, Cold War and American State: Lloyd V. Berkner and the Balance of Professional Ideals. Routledge, 2000.
- Joseph Lade Pawsey and Ronald Newbold Bracewell, Radio Astronomy. Clarendon Press, 1955.
- Kristen Rohlfs, Thomas L Wilson, Tools of Radio Astronomy. Springer 2003.
- D.T. Wilkinson and P.J.E. Peebles, Serendipitous Discoveries in Radio Astronomy. Green Bank, WV: National Radio Astronomy Observatory, 1983.
- Woodruff T. Sullivan III, The Early Years of Radio Astronomy: Reflections Fifty Years after Jansky's Discovery. Cambridge, England: Cambridge University Press, 1984.
- Woodruff T. Sullivan III, Cosmic Noise: A History of Early Radio Astronomy. Cambridge University Press, 2009.
- Woodruff T. Sullivan III, Classics in Radio Astronomy. Reidel Publishing Company, Dordrecht, 1982.
External links

- nrao.edu National Radio Astronomy Observatory
- The History of Radio Astronomy * Reber Radio Telescope – National Park Services
- Radio Telescope Developed – a brief history from NASA Goddard Space Flight Center
- Society of Amateur Radio Astronomers
- Visualization of Radio Telescope Data Using Google Earth
- UnwantedEmissions.com A general reference for radio spectrum allocations, including radio astronomy.
- Improving Radio Astronomy Images by Array Processing Archived 2011-04-04 at the Wayback Machine
- What is Radio Astronomy – Radioastrolab